Potable Water Reuse Report
Published by the University of Southern California ReWater Center in collaboration with Trussell
Series 2, Issue 2
12 March 2025
Pathogen Reduction Crediting for Reverse Osmosis: Getting Credit where Credit is Due with Good Surrogates
Key Takeaways
- Reverse osmosis (RO) is an excellent barrier against pathogens (e.g., 6-log10 virus reduction), but is under-credited for pathogen reduction due to the lack of good surrogates.
- Conductivity and total organic carbon, the most common surrogates, only provide log reduction credits of 1.5 to 2 log10. Other naturally occurring ions provide opportunity to increase RO credits up to 3-log10 .
- Alternative approaches—such as the use of spiked surrogates—have the potential to provide 4- or 5-log10, but more research is needed for implementation.
- This issue shows practitioners and regulators how to extend RO crediting with existing surrogates and points to the need for further research to maximize RO’s full potential.
1) Introduction
No single treatment process provides as broad control of pathogens and chemicals as reverse osmosis (RO). Pressure is used to force water through a membrane with nanoscopic pores that prevent the passage of most other compounds. The pores are so small that they restrict the passage of salts, pharmaceuticals, and pathogens. Not surprisingly, RO is used in many potable reuse treatment trains throughout the world.
Given that RO can remove even the smallest dissolved compounds in water (like the Na+ and Cl- in table salt), it may not be surprising that it is even more effective at removing viruses, which are relatively huge (approximately a hundred times larger than salt ions). Why then, does the RO treatment process receive a meager 1.5 to 2.0 log reduction value (LRV) credit for viruses when studies have shown that RO can reject up to 6 log10 of viruses? The answer goes back to a concept discussed in the last issue: it’s not about what you can remove, but what you can prove (see Series 2, Issue 1).
RO is not credited by directly measuring virus rejection, but by measuring surrogate parameters that correlate with virus rejection and are more easily measured. To bridge the gap between actual virus rejection and credited virus rejection, better surrogate frameworks are needed. In this issue, we explore what makes a good surrogate and why new surrogates are needed to ensure that RO gets its rightful credits.
2) RO is an excellent pathogen barrier
RO membranes consist of a thin (~0.2-µm) polyamide layer mounted on a thicker (40-µm) ultrafiltration membrane, supported by backing material. The top (polyamide) layer separates H2O from other constituents as feedwater is forced through the membrane’s network of interconnected pores ranging in size from 0.4 to 0.9 nm. RO membrane rejection is driven by size exclusion and electrostatic repulsion, so larger constituents (for example viruses) and constituents with higher charges (for example SO42- and Sr2+) are rejected better than smaller, uncharged molecules like water (Fig. 1).
Of the reference pathogens, viruses are roughly 100x smaller than both Giardia and Cryptosporidium, and yet viruses are still much larger than the RO membrane pores. For example, norovirus and MS2—the most common laboratory viruses used for RO challenge testing—are among the smallest viruses in water, and yet they are still 10x larger than the RO pores (Fig. 1). RO systems that have not been compromised (i.e., have full integrity) should easily reject viruses due to size exclusion, and studies confirm that uncompromised systems reject viruses by 6-log10 or more. Larger pathogens (e.g., Giardia and Cryptosporidium) should have even greater removal, but are typically assigned the same LRV credit as viruses.
Even though viruses are so much larger than RO pores, they are not entirely (100%) removed. Several mechanisms can lead to loss of RO integrity, including (1) deterioration of the O-rings that are critical for separating untreated water from treated water, (2) damage to the RO membrane surfaces themselves, and (3) leaks in the glue lines that bind the spiral-wound membranes together. Most of these issues, however, only lead to minor losses in virus removal efficiency. Major losses in virus removal efficiency only occur when integrity is severely compromised, such as when multiple O-rings are badly damaged or missing entirely.
Figure 1: The main challenge with RO crediting stems from the difference between pathogens and the surrogates used to track their removal. a) Many common surrogates are highly conservative because they are orders of magnitude smaller than pathogens, including viruses. The average diameter of norovirus is 27 nm whereas RO pore sizes range from 0.4 to 0.9 nm; b) While different surrogates are rejected to different extents by RO membranes, all commonly used surrogates underestimate virus rejection.
3) Better surrogates are needed to show higher log reduction values
The last issue described the three steps for developing pathogen crediting frameworks: 1) understand the mechanisms for pathogen reduction and how they are influenced by environmental, design, and operational factors; 2) identify surrogates that can be continuously measured and conservatively linked to pathogen reduction; and 3) establish an operational monitoring strategy with critical control limits to assign daily LRV credits. The US EPA created a crediting framework for the broad category of membrane filters called the Membrane Filtration Guidance Manual (MFGM) that requires both direct and indirect testing of membrane integrity. To date, this crediting framework has been better adapted for lower-pressure applications like microfiltration (MF) and ultrafiltration (UF). The reason for this is that the integrity of MF and UF systems can be tested directly using pressure (or vacuum) decay tests. The results of these tests can be used to quantify and then assign pathogen LRVs. Because a pressure decay test typically only occurs once per day, online measurement of a surrogate (e.g., turbidity) is required to provide continuous, but indirect, confirmation of the system’s integrity.
Currently, there are two important limitations with existing crediting frameworks. The first is that credit can only be obtained for the larger reference pathogens (i.e., Giardia and Cryptosporidium). This stems from the fact that the pressure required to confirm pathogen reduction is inversely proportional to the size of the pathogen. As a result, it is not yet possible to assign virus credits through pressure decay testing. The second major limitation is that RO systems cannot undergo direct integrity testing using pressure decay tests. Consequently, RO requires alternative methods for direct integrity testing.
One method is to track the rejection of a specific compound through RO. The most obvious compound to track would be a pathogenic virus. However, the impracticality of testing viruses on a daily basis precludes this approach. The alternative is to track the rejection of a surrogate. A surrogate is a constituent present in water that is easily measurable and that correlates with pathogen reduction through treatment. Ideally, a surrogate shows high LRVs for uncompromised RO systems and tracks with decreased pathogen removal if the system is compromised (Fig. 2).
Figure 2: The best surrogates will mirror virus removal in uncompromised and compromised reverse osmosis modules.
If surrogate-based approaches comply with the existing crediting frameworks, then why is RO under-credited? The answer is that we have not yet identified surrogates that track the full extent of pathogen rejection by RO. One key challenge for the reuse industry, therefore, is to identify surrogates that give RO its full due credit for removing pathogens.
Conductivity and/or total organic carbon (TOC) are often used as surrogates to quantify pathogen rejection by RO membranes. The first question to evaluate their appropriateness as surrogates is: do these parameters look and behave like viruses? From many perspectives these are not ideal surrogates. The constituents comprising conductivity and TOC are typically orders of magnitude smaller than viruses which allows them to partially pass through even uncompromised RO membranes. Consequently, intact RO systems generally show ~97% to 99% (1.5-2 log10) removal of conductivity and TOC at best. The use of these surrogates is the main reason that most RO processes in potable reuse systems are limited to 1.5- to 2-log10 units. Furthermore, they do not satisfy the definition for direct integrity monitoring since they are bulk parameters rather than specific compounds. They are still valuable as indirect measures of integrity, however, with both benefitting from online meters that can continuously track RO performance.
The issues with conductivity and TOC highlight the larger issue with RO crediting: the difficulty of finding a surrogate that behaves like a virus frequently leads to under-crediting of RO. An ideal alternative surrogate would have similar LRVs as viruses under compromised and uncompromised conditions as shown in Fig. 2, and would be:
- Naturally occurring in the RO feedwater at high enough concentrations to measure up to 6-log10 reduction and avoid the need for spiking,
- An individual compound; not a bulk water quality parameter,
- Rejected by uncompromised RO systems to a similar degree as actual viruses,
- Measurable with continuous, online monitors that are both easy to operate and inexpensive.
4) Alternative crediting strategies
Considerable effort has been dedicated to identifying alternative surrogates and ways to credit RO systems (Table 1). Overall, three approaches have been pursued.
Approach 1 involves calculating the LRV credit based on feed and permeate concentrations of a variety of surrogates that provide advantages over TOC and conductivity. Ideally, a naturally occurring, individual compound is identified that can be continuously measured to demonstrate the maximum virus reduction of 6 LRVs. This ideal surrogate is listed as “UNICORN” in Table 1. To date, the most promising alternative surrogates have been multivalent ions such as sulfate (SO42-), strontium (Sr2+), calcium (Ca2+), and magnesium (Mg2+), which generally show LRVs of ~3 (Table 1). However, one challenge is that the online analyzers used to measure specific compounds are typically expensive and require highly trained lab staff. Without online analyzers, grab samples have to be analyzed daily at a laboratory, which also increases cost and complexity. The benefit is higher LRV credits (i.e., 3-log10 instead of 1.5-log10). While this doubles the amount of credit received, it is still 3-log10 lower than the 6-log10 reduction of viruses. To date, there are no known surrogates present in the RO feed of potable reuse systems that are consistently removed by more than 3-log10. Given that this approach has gained regulatory approval for constituents like Sr2+, SO42-, this might be the easiest entry point for utilities looking to increase their RO pathogen credits without investing in additional research.
Approach 2 uses surrogates that are spiked into the RO feedwater to provide greater sensitivity to measure LRVs through RO. The best of these “marker-based” surrogates are ones that are highly rejected with LRVs most similar to viruses. Similar to Approach 1, credit is based on the difference between feed and permeate concentrations. Uncharged constituents (e.g., sucrose) and fluorescent markers (e.g., 3D TRASAR) have emerged as potentially useful alternative surrogates that can provide higher LRVs (~2.5 to 3) while also being measurable with online sensors. The main challenges of spiked surrogates are the cost and complexity to spike in the compounds and unresolved questions about the effect of the compounds on membrane fouling. As such, this approach has not been used in full-scale potable reuse projects and has not received regulatory approval (Table 1). Theoretically, a spiked surrogate has a higher LRV ceiling than the use of naturally present surrogates if researchers can identify a compound that more closely matches virus LRVs.
Approach 3 uses modeling to develop relationships that link the high rejection of viruses with the more moderate rejection of surrogates (e.g., conductivity). Through such modeling, an RO system that achieves 1.5-log10 reduction of conductivity could seek credits above 1.5-log10 for virus. The use of modeling is different from the first two approaches, which calculate LRVs directly from the surrogate concentrations. Key to the modeling approach is the implementation of a robust integrity monitoring program to identify breaches that would allow increased virus passage. Daily conductivity profiles, which require monitoring of the permeate of each vessel of an RO system coupled with the use of the dilution model approach described in the MFGM, has been proposed as a way to assess integrity at the individual vessel level. While the theoretical ceiling of this approach is high, more research is required to acquire regulatory approval.
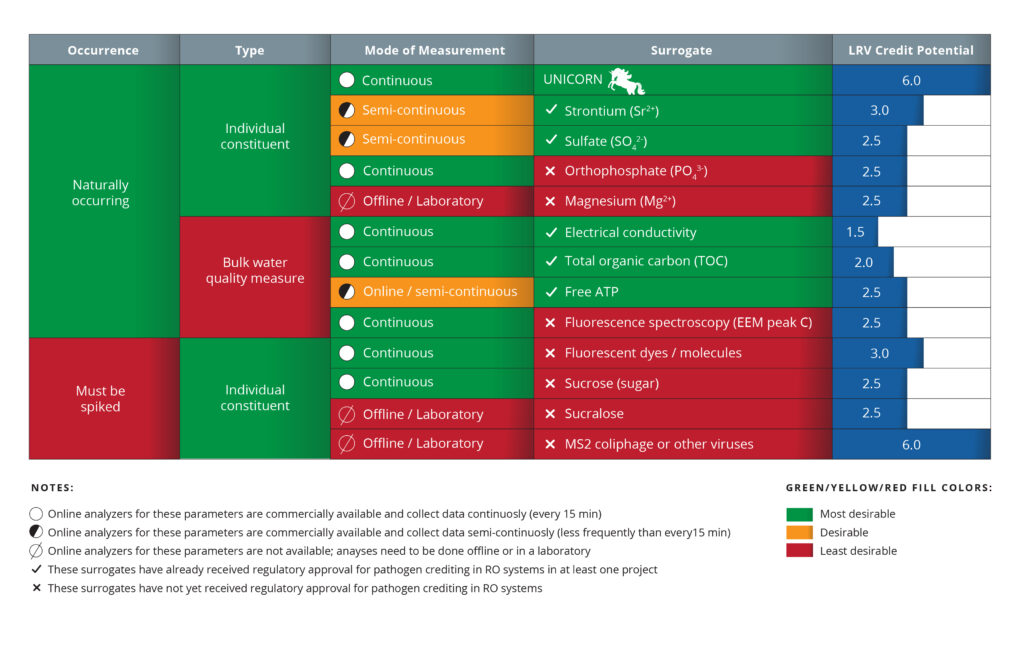
Table 1: Current and emerging surrogates and integrity testing methods for pathogen crediting in RO systems.
5) Carving a path forward to get credit where credit is due
Currently, at least 1-log10 of additional credit can be gained by switching from conductivity to strontium, sulfate, or other multivalent ionic compounds (Fig 3). This approach has already received regulatory approval on several projects in Southern California. With more research and further development of integrity monitoring approaches, it may be possible to get up to 5 or 6 log10 credit for pathogen reduction in RO systems (see “Remaining gap” in Fig. 3).
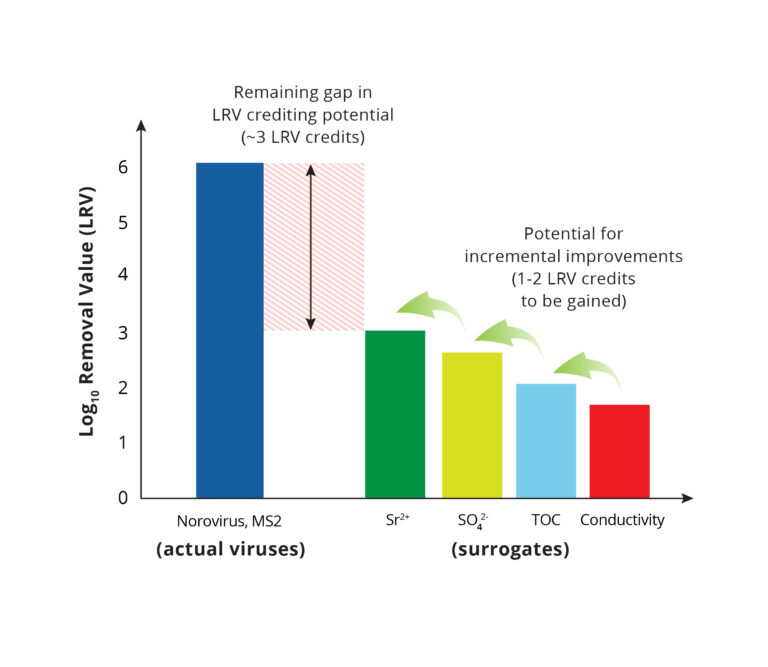
Figure 4: Potential for reducing and eventually eliminating the gap in virus reduction crediting for RO systems.
Before this can happen, however, some outstanding questions need to be addressed. First, there may be a need to revisit and confirm the definition of direct and indirect integrity testing for membrane systems. Bulk measures of water quality like turbidity, TOC, and conductivity are defined as indirect approaches for integrity monitoring in the MFGM. Experts in the field have argued that daily measurement of specific constituents, such as sulfate or strontium, meet the MFGM definition of direct integrity testing and therefore can be used to assign pathogen credits through RO. This understanding of the crediting framework should be confirmed with regulators.
There are also outstanding questions about the resolution of monitoring needed for compliance. Full-scale RO systems typically include multiple trains with each train being a collection of multiple pressure vessels. What level of granularity is needed for monitoring? Should individual pressure vessels be monitored, or is it sufficient to monitor an entire train? Or, is it sufficient to monitor the overall feed and the combined permeate for the entire system?
For most indirect potable reuse projects, the combined permeate has been monitored, with LRV credits assigned based on the reduction of highly conservative bulk surrogates such as conductivity. As potable reuse projects attempt to get higher, more representative LRV credits using alternative surrogates, there may be a shift toward monitoring RO systems at a higher resolution (e.g., individual trains or individual vessels). This shift would bring clear cost and complexity tradeoffs, including the cost for additional online meters to monitor the permeate at more locations.
The lack of clarity regarding how to credit RO has led to inconsistency in the way RO is credited from one location to the next. For example, for potable reuse projects in Texas, the RO process currently receives no credit for pathogen control. California has traditionally relied on bulk parameters for crediting RO in indirect potable reuse but is moving toward requirements that are more in line with the MFGM for direct potable reuse. Moving forward, one option to provide more consistent implementation across the US is to update the MFGM to include guidance that is specific to RO. Such guidance would benefit potable reuse applications while being transferable to industrial and other forms of reuse.
Beyond RO, there are multiple uncredited processes that have no crediting frameworks. For example, membrane filtration offers no virus credits, and both granular media filtration and wastewater treatment offer no default credits for the reference pathogens (virus, Giardia, or Cryptosporidium). Issue 3 of this series will describe how a preliminary crediting framework has been developed from scratch for the membrane bioreactor (MBR) process, and how that can serve as an example for the future development of frameworks for other uncredited treatment processes.
Sign up for our email list to receive the Potable Water Reuse Report
The contents of this report are not to be used for advertising, publication, or promotional purposes. Citation of trade names does not constitute an official endorsement or approval of the use of such commercial products by the US Government.
All product names and trademarks cited are the property of their respective owners, the findings of this report are the opinions of the authors only and are not to be constructed as the positions of the US Army Corps of Engineers or the US Government unless so designated by other authorized US Government Documents.